Using Cutting-Edge Computation to Understand the Foundation's of Life's Mechanisms
Nature has evolved a wide range of mechanisms to harness the physical and chemical properties of biological molecules to perform essential biological processes. Advances in biophysical theory, experiments, and computing have created unprecedented opportunities for studying these foundations at the atomic-scale. Working in close collaboration with our experimental collaborators, our group utilizes and develops computational tools to study diverse biomolecular mechanisms and complexes. Some of these projects include:
Molecular Mechanisms of Epigenetic Regulation
The blueprints for life are written in the genomes of all organisms. However, to recognize the potential in this code, genes must be selectively expressed and repressed in response to external stimuli and non-genetic inheritance. At the molecular level, these epigenetic processes are controlled through multiple mechanisms. In eukaryotes, DNA is packaged in a hierarchy of structures that involves organizing approximately equal amounts of protein and DNA molecules into chromatin fibers, with the most basic unit being the nucleosome core particle. We are interested in understanding the processes by which DNA is compacted into chromatin fibers, and how the cell can alter the physical and chemical properties of the genome to control gene expression via epigenetic regulation.
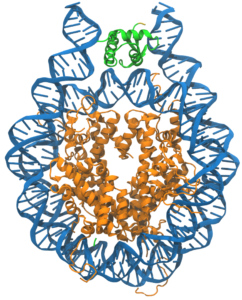
DNA (blue) is compacted by binding to eight core histones (orange), and further compacted through binding a linker histone (green) (adopted from Woods and Wereszczynski).
Molecular Underpinnings of Bacteria Virulence Pathway
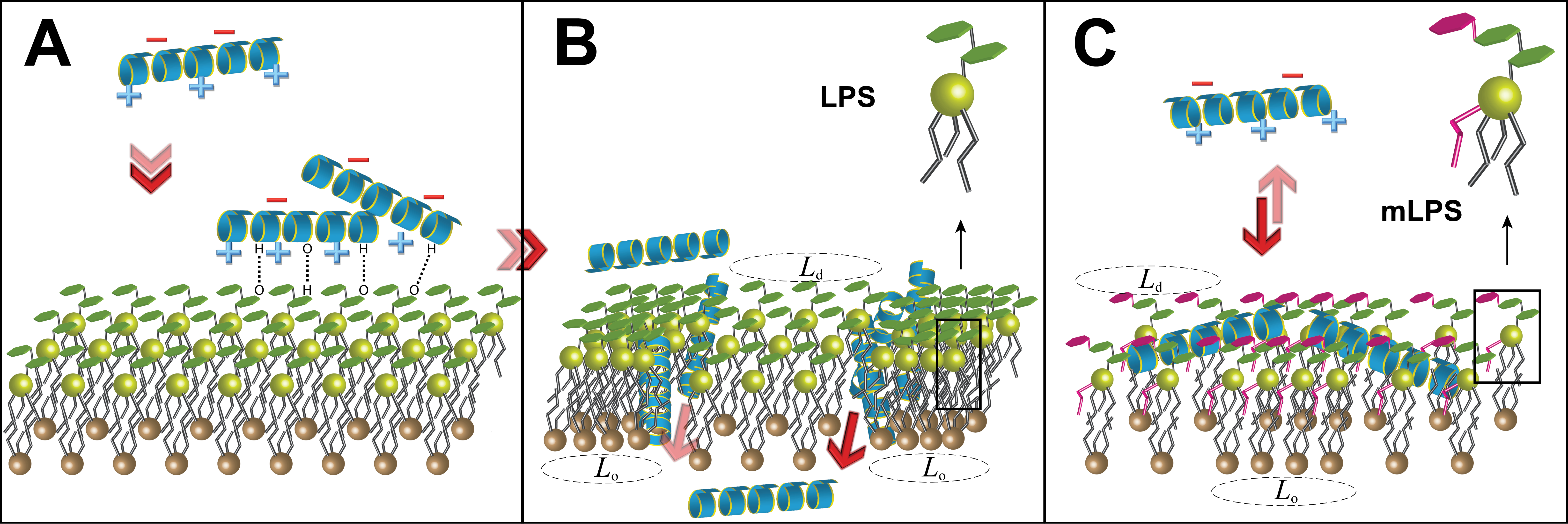
Mechanism of action of the antimicrobial peptide LL-37 against standard, LPS-containing, and modified LPS-containing bilayers (from Martynowycz et al.)
The rise of antibiotic-resistant pathogens is a leading threat to global public health. One potential avenue for the development of next-generation antibiotics is to target virulence factors: pathways that are not vital to bacterial survival but that instead aid the bacteria in invading and colonizing hosts. Indeed, it is hypothesized that by not disrupting the growth cycle of bacteria, antibiotic resistance may spread significantly slower through a population with decreased virulence, as resistant strains will be forced to compete for resources with bacteria that are effectively “disarmed". However, in most cases, it is not clear how virulence pathways function on the molecular level, or how they may be disrupted by medications or the natural immune response. Therefore, it is imperative that we understand the molecular underpinnings of these processes to determine how to rationally proceed with the development of antivirulence compounds. Our group is working with experimentalists around the country to examine various facets of bacterial virulence factors, and how they can be disrupted by natural and synthetic molecules.
Robust Modelling of Small Angle Scattering Experiments
Small angle X-ray scattering (SAXS) is an invaluable tool for studying the structure/function relationship in biomolecular complexes. It has several advantages over other structural techniques, including the ability to perform experiments on a wide range of targets, in near-physiological conditions, and to provide structural data for disordered regions of proteins. However, the advantages of SAXS come at a cost: the scattering data generated in experiments is of low-resolution and provides limited direct information concerning the tertiary and quaternary structures of single molecules and biomolecular complexes. Simulations can, in theory, provide the “missing link" that connects measured scattering profiles to atomic-scale structures. Historically this approach has been limited by the physical models in use, the time and length scales that can be simulated, and the cumbersome nature of most modeling software packages. Therefore, one of the grand challenges facing the field remains the development of methods that effectively utilize the scattering profiles measured in experiments to interpret the relationship between the structure, function, and dynamics of biomolecules.

Fitting of conventional and accelerated molecular dynamcis simulations results to experimental SAXS data (from Bowerman et al.).